How to shoot down a target in space? The answers will be different even for different specialists: everyone sees their sides of the case. Can we, for example, look at interception in space through the eyes of a flight dynamics and motion control specialist? It is a kind of flight designer and developer, an architect of movement and flight situations. According to his flight scheme, metal designers work, creating the device, and launch teams. The means of space interception will also fly according to his decisions.
In this case, we would see the task from the side of flight dynamics and control, and this is a very interesting view. Simplifying a lot of things, with flaws, it is quite possible to do this.
The technical system looks different through the eyes of the developer than for others. The creator sees in it, first of all, a tangle of meanings fulfilled in the design. This is how he perceives the tasks he is looking for solutions to. He is also armed with knowledge from special fields, often closed to the masses. Let's try to look at the object with his eyes, trying on his train of thought and logic.
Beyond the atmosphere: the edge of heights and speeds. What kind of goals will be in it?
The formal boundary of the atmosphere is assigned a height of 100 kilometers, and above it lies space. All objects flying there move according to the laws of ballistics, with little influence of aerodynamic forces. Their movement features are grouped by height and speed. Low-orbit satellites and warheads of intercontinental missiles at extreme ranges form a high-altitude and high-speed segment of targets: their speeds lie in the range of 6.0-7.5 kilometers per second.
The lower segment is formed by medium-range and tactical missiles, as well as multiple launch rocket systems with a range of 200 kilometers or more — their rockets can also rise above 100 kilometers. The flow is also important, taking off even higher, including ICBMs flying already above 100 kilometers, and their combat stages of breeding with warheads. They differ in speeds (which are growing fast). Immediately after the atmosphere, these targets have not yet accelerated much and have not gone high, they can be attributed to the lower segment.
It consists entirely of elements of weapons — warheads and combat missiles of different classes. And it grows faster over the years than the top one, because it is simpler and the first to be mastered when spreading missile technologies to all new countries. It can be used to guide the selection of typical goals.
This is how the speed and height of a typical space interception target are outlined. Their heights are the first hundreds to a thousand kilometers, the distance (the distance to the target in a straight line) is a couple of times greater, and the speed is two to five kilometers per second. The atmosphere no longer has a tangible effect on them: it is too high. Targets can maneuver in the void several times and be in groups.
With the development of technology, a ballistic target will be able to actively evade an attacking interceptor missile, dynamically changing its trajectory based on the actions of this very interceptor. It will turn out to be a kind of hybrid of a ballistic warhead and an anti—missile, which will engage in a duel, but not with the task of shooting down another missile, as with a conventional anti-missile, but with the opposite - the task of avoiding interception. It is as well solved in the acrobatics of rocket combat as the chase. But so far, such advanced systems are the future. Today, the maneuver of a ballistic target is implemented simply by several inclusions of the block of lateral solid-fuel maneuvering engines.
Interception is the forced termination of a target's flight in the same direction: destruction or deviation of the trajectory. You can destroy a target in space with different "physics". With a laser, although there are no such real combat systems yet. It is possible to make a nuclear charge non-explosive by irradiating its plutonium with a dense neutron flux. This will critically enhance the initial level of nuclear reactions in plutonium and, instead of an explosion, will give a premature "pop"; but this is in theory (will it be an interception if the trajectory of the target does not change in any way with a "rotten" charge?).
The real method of defeat, which is already working today, is different: a mechanical blow. Only a rocket can deliver such a strike quickly and accurately. It is the missile interception that is the base of operations in lower space.
Than to hit the target when they flew up
The shock wave of a high-explosive charge is not suitable for interception either in space or in the atmosphere. In space, it will quickly weaken with the spread of gas, and in the atmosphere it will go through still air, also quickly dissipating (a supersonic target may simply fly away from it). The kinetic impact of a material body works to defeat in such conditions.
Fragmentation defeat is a classic interception. A cone or stream of fragments hits the target and pierces it, critically disrupting its functioning. Ready-made striking elements make it possible to form a fragmentation field more accurately and efficiently. Controlled fragmentation fields are a very effective, interesting and developed topic, but about it another time.
Their continuation, the core warhead, opens a grid of steel rods with a slight explosion and "cuts" the target with it from the raid. The same logic is continued by the kinetic warhead — a direct hit to the target, but without a grid of rods, just with the body. So a battering ram suddenly came into space from aviation, remaining an effective and modern method of destruction already at the rocket and space level. Performed according to the principle of "shoot down a bullet with a bullet" in an autonomous mode by a small-sized self-guided ramming device. And it has advantages that make such a choice an effective solution.
The size and mass of a kinetic interceptor can be small, and a kilogram is enough to defeat it. A counter strike at a speed of five to eight kilometers per second does not require explosives, acting on the target with greater force. We do not need a combat unit, a detonation system, a link in the development of a combat team for detonation, a battery. There is no need to determine the moment of detonation. The entire on-board kitchen and filling come down to one thing: combining your trajectory with the goal before the final meeting. This is called guidance.
Guidance through the desired behavior
There are many types of guidance. Several homing methods, several command guidance options, autonomous inertial, with astrocorrection (by stars) or GPS correction — all this can work on board our device.
The guidance process is conducted by the guidance system included in the flight control system. Like any technical system, it operates with an error, while the current miss for a kinetic interceptor should be less than the size of the target. That is, less than a meter.
The essence of guidance is in turning the trajectory. Continuous or in pulses with frequency and quantity in series, strong or small, it bends the trajectory. The bending force is the thrust of the maneuvering jet engines. For bending, the lateral overload created by the thrust is important, which will determine the rotation parameters: direction, radius and time.
Flight dynamics-1
Overload is acceleration expressed in g, the average acceleration of gravity on the Earth's surface. It is intuitively more convenient in technology, and is widely used in it. A tank weighing a ton in a five-fold overload weighs five tons, the design should "hold" this weight. Therefore, in rocket science, they like to operate with overload and its values.
What is the difference between a rocket and a tram? The tram rails at the turn are laid curved in advance. Going sideways, they put pressure on the tram, leading it to the side and creating a lateral overload, pulling, respectively, sideways. It turns out that for a rocket, the cause and effect of maneuvers are the opposite of tram maneuvers. A lateral overload is created on board the rocket, which bends the trajectory into a turn. And it comes out the way the overload is: as it increases, it decreases the radius and turn time. The trajectory of the rocket turns due to the lateral overload created on board.
Atmospheric rockets (anti—aircraft, air-to-air and others) create a huge lateral overload up to 40-90 g for the sake of maneuverability. Their hull is pushed sideways by the powerful pressure forces of the compressed air flow (the rocket is placed in it at an angle of attack).. There are no external pressures in space, no matter how you turn the case; and no less overloads are needed. Only jet engines of maneuvering work there. Several nozzles on different sides of the device create a lateral thrust force. Groups of engines of different power are also possible: powerful ones turn harder and faster, weak ones more precisely.
Overload does not depend only on the engines. Reducing the mass of the device will increase the overload from the same thrust. After all, overload is acceleration, the ratio of engine thrust to the mass of the device. More overload means faster and steeper turns; the low weight of the device increases its maneuverability. This is how the idea arose of a light, compact and maneuverable kinetic interceptor separate from the rocket, operating on the final leg of the flight to the target beyond the atmosphere. The rocket lifts it into space and accelerates it directly to the target, after which it separates. Further flight is the independent work of the interceptor in its high—precision movements. For the success of which he will need a lot.
Where is the goal? Find her more precisely
For example, you need data about the target — where it is and how it is moving. You can see the target in the radio, optical, ultraviolet or infrared channel. Optical does not work well in the shadow zone of the Earth (it is dark). In radar, the good old rule applies: the more powerful the radiation, the more accurate the image. The power of the radio beam will determine the target detection range. However, the mass of the transmitter and batteries is growing, reducing the lateral overload and maneuverability of the interceptor.
It is possible to transfer the radiating power and mass to the upper stage of the rocket. Beyond the atmosphere, it flies in free flight in the same way as the interceptor, braking equally in the local gravitational field equal for both. Therefore, the rocket stage is flying somewhere nearby with an interceptor. You can place a target illumination radar on it, and the reflected signal will be received by the interceptor. The receiver will take up a minimum of mass compared to the emitter and its power supply. But this will require a long, pre-interception, orientation of the rocket stage to the target. It is easier to irradiate a target with a much more powerful ground-based or ship-based radar.
The infrared channel is reliable enough. The task of thermal observation of individual dim targets in space is not always simple, but it is being solved. The torches of the ICBM upper stages are quite bright in this range. The engines of the combat stage of breeding can run on compressed carbon dioxide or nitrogen, without giving fire, thermal brightness and visibility. But the stage body will not have time to cool down after launch, and it will be nice to glow at its temperature in the infrared range. And the thermal sensor can be cooled with liquid nitrogen to improve the quality of vision. Infrared homing heads are compact, lightweight, accurate and sensitive; they are quite suitable for the role of the "eye" of an interceptor missile.
Kalman Filter: Mathematics for Apollo flights
Based on the data on the positions and speeds of the interceptor and the target, the process of approaching and controlling the movement of the interceptor is being built. Algorithms for data analysis and generation of control commands use special mathematics. For example, the Kalman filter is a system of differential equations for calculating a posteriori (i.e., based on actual observations) probability density and a set of values of mathematical expectations. As a result of its operation, it is possible to obtain an optimal (that is, with a minimum standard error) and continuously updated estimate of the position and velocity vector of the target in conditions when the target is poorly visible: indistinctly, in interference, or intermittently.
There will always be interference in any observation channel, regardless of the type of channel, because the universe is not empty. In addition to the target, there will always be something else visible in this channel in the field of view. Optical, thermal, radar, acoustic, seismic, gravimetric, any. The world is visible in any observation channel, and the goal is visible in it embedded in the background of everything else being observed. Noisy, clouded, illuminated, colored, shaded, blurred, muffled: interference lives everywhere and is infinitely diverse.
In addition, the exact values of the coordinates can be "blurred" by the strong brilliance of the target itself: a clean background, the absence of observable other things, can make the target look "brighter". Then the blurring of the target with its great brilliance will compensate for the absence of interference from a clean background. And the "eye" itself will also give its interference, no matter how structurally it is. And there will be even more interference in the case of his not quite correct work. Clarification of the position of the target, observed with all these difficulties, can be given by Kalman filtering.
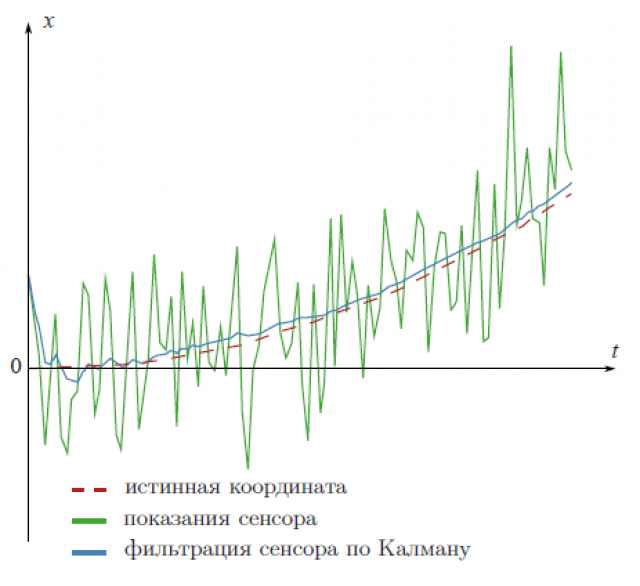
A scheme for clarifying observations of the X coordinate by the Kalman filter during time t. Source: habr.com .
But this filter can clarify not only the current position and speed, but also their most correct (optimal) forecasts for future moments of time. That is, it is able to predict the most likely trajectory of the target with the least error.
These forecasts are continuously refined by a two—step filter algorithm based on current observations (extrapolation - refinement). The Kalman filter is just one example; it can be supplemented with a mathematical linear-quadratic regulator (with which the Kalman filter works), and others. We will not delve further into mathematical terms; we will leave Gaussian control, the variance matrix, the dynamics of the state vector and the estimation of its distribution density, and much more, with all the understandable interest in them.
All this is by no means the development of the XIX century. Rudolf Kalman proposed and incorporated his filter into the computer navigation system of the manned Apollo spacecraft. Wikipedia will remind you: "Kalman filters have proven to be critically important for the implementation of navigation systems of US Navy submarines with nuclear ballistic missiles on board, in cruise missile navigation systems, for example, Tomahawks. It was also used in the navigation and control systems of the NASA Space Shuttle project, and is used in the ISS control and navigation systems."
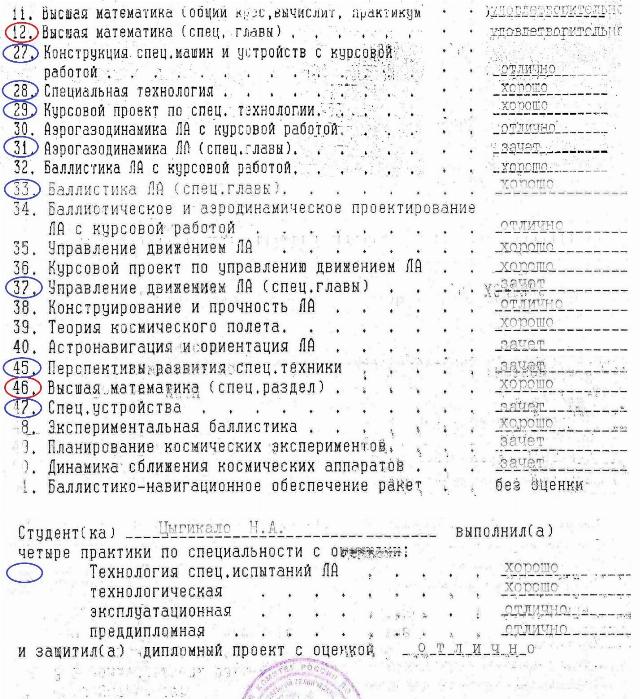
An example of special higher mathematics in the diploma extract, marked in red. And in blue there are 9 other "specials", a total of 11 items "special" and "specials", often meaning classified information. Some of the numbers were skipped in order to squeeze the necessary two pages into one picture. From the author's archive
Sections and chapters of higher mathematics with such special content are very interesting, especially developing areas. Most of this mathematics is not openly published, some of it is classified.
Such areas of mathematics are called special chapters and special departments of higher mathematics in specialized organizations (see photo). Approximately the same math will work on the interceptor.
A difficult TCB will pave the way to heaven
In addition to special mathematics, there is an important and rich theory called "management in technical systems", or TCB. She sees a technical system as a group of interconnected links that include a management object. The main idea of TCB is the reaction of a link to a signal or command of a unit value.
Having received an impact with a sharp step in one unit, the link reacts to it — after a while (reaction time) it goes into a new steady state. The transition can vary greatly for different links. For some, it will go on a long gentle rise to a steady level. For others, the state will change with a fast, steep and "off-scale" surge, which will rise like a high hump of a wave, and then gradually decrease to a steady new state.
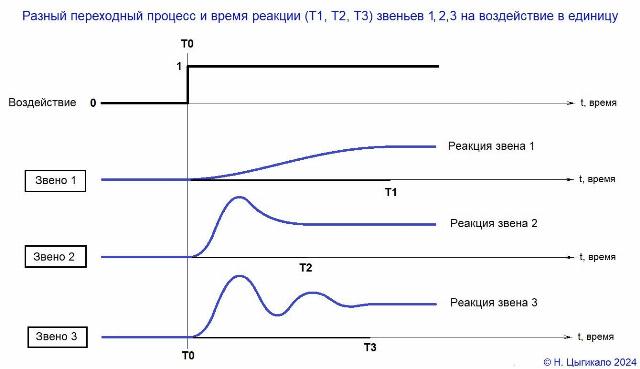
Both links will pass (each in its own time) through their transitional states, one growing for a long time to a steady state, the other immediately exceeding it. And there may be damping fluctuations on the way to a steady state. And non—extinguishing - the properties of a link and its behavior after exposure to an incoming unit can be very different. And by giving commands in several units, we get the reaction of the link to the control command in a wide operating range.
Several sequentially or parallel connected links will give the output reaction of the last link, usually the control object. Its magnitude and the nature of the increase in this reaction — that is, the behavior of the control object — can be determined by mathematical rules and formulas describing patterns in this area. You can add feedback from any link in the chain to any previous one, making it negative or positive. This looping will complicate the reaction (and change its time) of the control object to the effects on the links of the system. There are several, or even many, feedbacks from different links in the chain to different preceding links, up to dense wilds. As well as several "entrances" in different places.
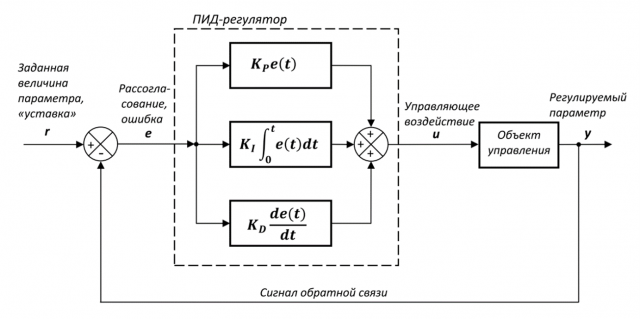
An example of a system with a proportional-integral-differential regulator (PID controller) containing three links (proportional, integral and differential from top to bottom, this can be seen in each link according to the formula describing the behavior of the link), a control object and negative feedback.
Such a link system can also be declared a link with its own complex properties. His reaction to the incoming impact is set by rules and formulas, so it is natural and can be accurately calculated. The behavior of a link may have new characteristics — for example, it may be stable or unstable; or it may be stable up to one level of incoming impact, and unstable above this level. Instability means the absence of steady-state end states, the reaction of the link "goes awry" in one form or another. As a result, it does not work out to achieve the desired steady state of the system at the right time: management is ineffective. The transition from stability to instability is determined by special stability criteria of Lyapunov, Hurwitz, Raus, Mikhailov, Nyquist and others.
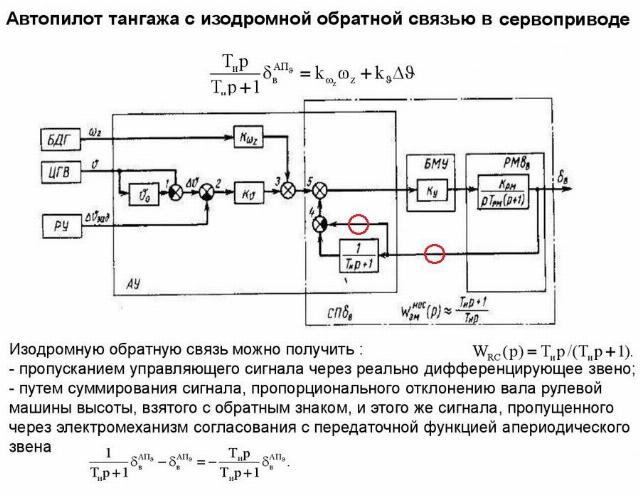
An example of a more complex pitch control system: more links, 3 inputs and two feedbacks (marked with red circles). In each link, its law of behavior or function is written. The upper formula expresses the law of behavior of the control object, the rightmost link (RMV). This is an altitude steering machine, a drive that sets the position of the aerodynamic surface or the angle of rotation of the nozzle, controlling the lifting of the nose of the rocket above or below the horizon.
There may be several tens or thousands of links, and the properties and behavior of a system of them are extremely complex, although calculated and known. Before the last link, a control action is formed that goes to the control object and brings it to the precisely calculated desired state. He also logs into the system, always connected to it by both incoming and feedback. By it, the object of control both affects and is present in the system, affecting its dynamics. And the system needs data from the control object to know its status and act correctly. Without feedback, management will go blind and become impossible.
In our case, the result of interceptor control will be its current orientation, magnitude and direction of velocity, trajectory, position relative to the target, parameters of approach to it, et cetera.
We'll leave the TCB at that, although we didn't even mention many important points. But otherwise there is a risk of never completing a brief examination of the principles of management theory. And although at first glance it seems to be an incomprehensible technical jungle, this is part of the interception process in a more detailed examination.
"Time, go ahead!"
To increase the accuracy of motion control, it is necessary to increase the speed of the flight control system links. This includes the choice of fast operating algorithms, and the speed of measuring systems, electronic filling, and the executive segment: jet engines and the working fluid supply system. It may take not a millisecond, but a microsecond (one millionth of a second) time scale in which the work of the links is built.
Microsecond time is not as simple as millisecond time. The latter is used in missile tests, it is given by the receiving points of the unified time system of PP-SEV, which supply millisecond time to all other recording equipment. The current millisecond time is always printed on a rotating scale on the side of each frame of the phototheodolite film of the FRS-2 optical trajectory measurement station operating on an optical target (for example, a warhead in the atmosphere). Without it, the stations will put a deuce on combat work, because the frame is not readable without an accurate time reference, the trajectory information is lost.
The microsecond scale is used in other cases where a large amount of allocated power leaves little time to control the process. Microseconds are used in controlling nuclear charges that require high precision of action. Such a time scale may also be needed to control the movement of the interceptor for the sake of guidance accuracy. Maximum performance will be especially necessary at significant angles of intersection of the trajectories of the target and the interceptor.
Interception angle: easy, difficult, difficult
Indeed, the angle of the interception, or the mutual directions of movement of the target and the interceptor, will determine its complexity. The simplest (and thus special) case is precisely a frontal approach. Then the trajectories of the target and the interceptor coincide in one common line, and the target is always directly ahead of the interceptor's flight path, occupying the same point in the sky and the center of the field of view. There is no need for maneuvering or calculating the moment of the meeting: sooner or later in this situation, a frontal impact is inevitable.
In reality, the trajectories of the target and the interceptor will intersect (of course, in case of successful guidance) at some angle. And it is important to ensure the simultaneous arrival of the target and the interceptor at a common point of intersection of the paths. This will require adjusting the speed not only in the direction (bends of the trajectory to create a common point), but also in magnitude, so as not to be late and not to miss the common point before the target.
As the target approaches, it shifts faster and faster into the interceptor's field of view, and the bending of its trajectory during homing increases: lateral overload increases, reaching maximum values by the time of the encounter. Depending on the guidance method, a final stretch without overloads is also possible. But only if this guidance is unmistakable; in reality, when approaching, the mutual movement is clarified, and interceptor corrections are necessary.
Hence the different probability of interception from different angles: on frontal courses, maneuvers can be reduced to a minimum, simplifying guidance and increasing the effectiveness of interception. And intercepting from the side is more difficult and reduces the likelihood of a hit. There is no guarantee that the interceptor will shoot down the target in any case. He will work out with the probability of hitting, which will be set by the situation of convergence and his actions. The probability should be sufficient to put the technical system into service.
Rocket: flying to the right moment
Which missile to launch the interceptor with? It is necessary to accelerate faster after the start so that a free ascent of hundreds of kilometers in the braking gravitational field leaves several kilometers per second of speed at the local altitude. And the goal is high-speed, there is little time to fly to it. The solid-fuel engine will provide starting overloads of 100 g, at the same time almost nullifying gravitational acceleration losses. Control of the rocket in the atmosphere is possible aerodynamically, through the flow of the hull and steering wings.
Beyond the stratosphere, the air is too thin for aerodynamic forces. The control will become gas-dynamic, using the rotation of the engine thrust, gas-dynamic rudders immersed in the flow from the nozzle of the main engine, deflection of a part of the jet stream, or small separate engines.
Missile control will be different from interceptor control. First of all, the fact that the missile will not fly immediately exactly to the target, as the interceptor later did, but to the calculated area, which can be many tens of kilometers away from the current position of the target.
Therefore, the missile will not use homing, for which it is necessary to continuously see the target (especially if it is still far away). The rocket uses command guidance, where control commands are generated by the ground (or ship) center and transmitted to the rocket board. This requires a communication channel, its equipment will increase the mass of the rocket, and the radio channel itself, with all signal ciphers, can be an interference input. You can combine missile guidance with a combination of inertial and command guidance, complementing astrocorrection, GPS, and more.
Flight dynamics — 2
The rocket must contain fuel for sufficient energy at interception altitude. But climbing to altitude and acceleration is not all; energy can also be spent on improving the accuracy of interception. To do this, we will add a fuel reserve for the rocket factory on a "more oncoming course"; at which, as we remember, the probability of interception is higher. Therefore, it is worthwhile to draw an arc in the sky with an approach to the center of the front hemisphere of the target, and this will significantly simplify the hit.
So the issue of improving interception can be solved energetically, with additional fuel to move the interceptor into the space in front of the target. And this is an effective trick of the flight path developers, which gives a combat win.
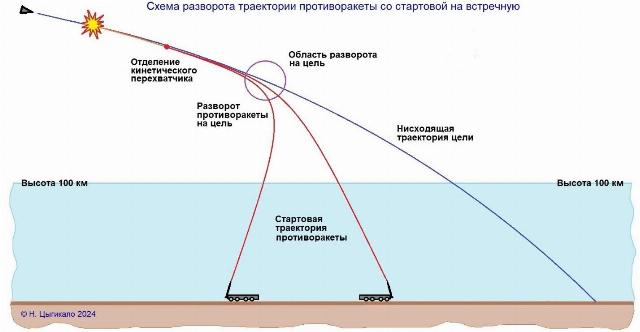
The optimal trajectory arc is not circular, but rather hyperbolic, with straightened sides, like a wooden yoke over a horse: there is a bend above the withers. It falls on the area of the turn towards the goal. You need to get there as soon as possible from the start, you will have to fly more straight.
The missile enters an area of space that lies well ahead of the target's movement and closer to its projected trajectory. There is a good counter angle with the target, and there the missile manages to turn towards the target and fly straight at it, soon separating the interceptor.
Examples of non-atmospheric warfare systems
The first missiles for trans-atmospheric interception flew there normally in energy, poorly in accuracy: their guidance, due to low accuracy, did not allow them to shoot down the target with fragments. Therefore, special (nuclear) combat units were placed on them. They compensated for the miss with a decent radius of nuclear destruction. The 51T6 Azov missile of the Soviet A-135 Amur complex with a maximum impact height of 670 kilometers had a length/ diameter of 20/2.5 meters and a mass of approximately 40 tons (averaging different data).
It was equipped with a thermonuclear warhead of the megaton class: thermonuclear power and its difference do not weigh so much, you can not save on them.
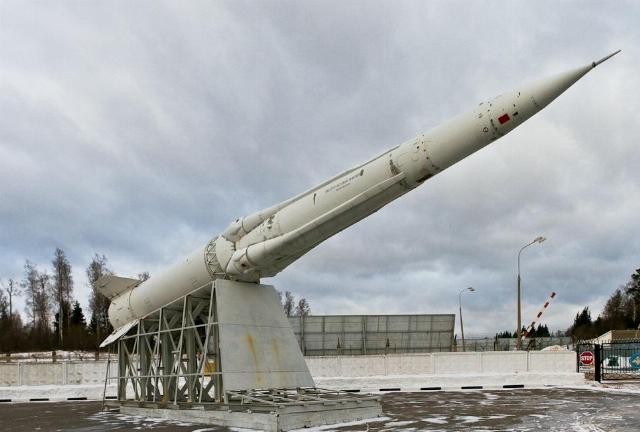
The 51T6 Azov missile of the Soviet A-135 Amur missile defense system. Photo: wikipedia
Time passed, and the three-stage SM-3 anti-missile (developed by Raytheon, which is in service with several countries) showed other characteristics: at a height of 1,500 kilometers and a length / diameter of 6.5 / 0.5 meters, it has a mass of only one and a half to two tons (in variations). The missile is fired at the target according to the commands of the ship-based complex, and does it in stages.
The first two stages accelerate the rocket and take it out of the atmosphere, setting it on a collision course with the target. The third stage engine operates in pulses for precise speed control and to ensure a meeting with the target. After its operation, a kinetic interceptor with infrared homing and receiving hints from the ship to search for a target is separated.
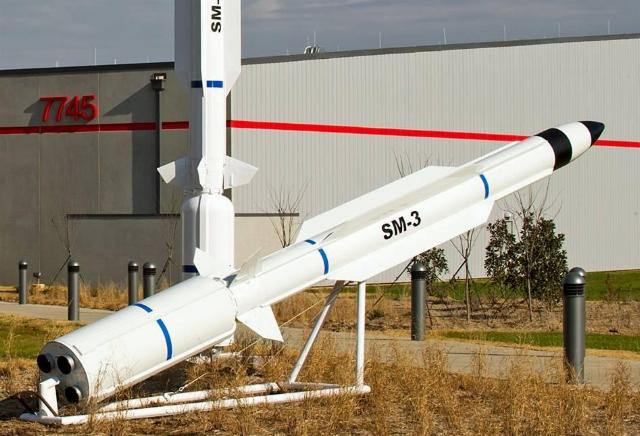
The SM-3 rocket. Photo: topwar.ru
It is equipped with a matrix dual-band thermal homing head with cryogenic cooling, detecting a target from a distance of 300 kilometers or more. During guidance, the interceptor can deviate up to 3-3.5 kilometers away from its initial trajectory. And it does this with the help of a solid-fuel propulsion system for maneuvering and orientation TDACS weighing only five kilograms.
Her work from the point of view of flight dynamics is interesting and eloquent. Let's describe it.
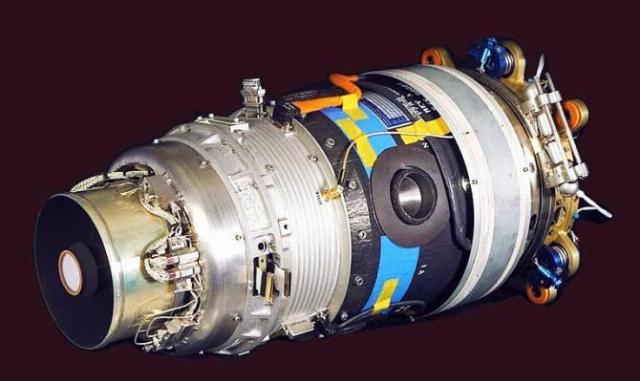
Kinetic interceptor LEAP missile SM-3. Photo: Forum avia.ru
A solid-fuel gas generator with several fuel charges produces gas of high temperature (2000 degrees Celsius) and pressure. High-speed valves bypass gas into the jet nozzle blocks at a frequency of 200 Hertz, that is, the nozzles work up to 200 times per second. This is the millisecond operating time of the end devices, a single nozzle operation in five milliseconds. This time is occupied by the final execution of commands by working organs, valves and nozzles.
Before that, there is a process of measuring the movement of the interceptor, the position of the target, the work of mathematics provided by several onboard processors and other actions. This entire cycle fits into five milliseconds, which indicates a much higher performance of on-board systems: they work at the microsecond time level.
The Russian A-235 Nudol transatmospheric interception complex, which replaces the A-135, is still being tested. Along with the upgraded 51T6 missiles already familiar to us, it will include other missiles capable of non-nuclear interception. Due to the secrecy, it is not yet possible to give their parameters and details of operation, like the SM-3.
Perhaps some parameters may exceed the American equivalent. More than a dozen successful test launches have already been conducted. In one of them, on November 15, 2021, the Kosmos-1408 satellite, which had spent its life, was destroyed at an altitude of about 650 kilometers.
Judging by the many final fragments of the satellite, it was a kinetic interceptor strike. Alas, this is a clogging of space; but if you just chop the target with fragments without breaking into pieces, radars will not be able to determine whether the target is hit or not. We will have to launch new missiles at it, before radar confirmation of the target's defeat through observation of its fragmentation. In breaking a target into pieces, another advantage of a kinetic interceptor is that such an action provides proof of defeat.
There are many more technical systems capable of intercepting in space than these three examples. We will leave their enumeration and comparative analysis because of the many details that would have to be delved into. Many of these systems are on combat duty, working today, sometimes setting combat records.
Recent news from the celestial empire
Israel conducted a combat interception at a record height on November 9, 2023. The Burkan-2 ballistic missile, a descendant of the Soviet Elbrus (Scud-B according to the Western classification), was launched from Yemen into Israel in combat mode. Its range reaches 1,000 kilometers, which classifies it as a medium-range missile.
The Israeli EL/M-2080 Green Pine radar detected the launch and transmitted the motion parameters to the Golden Citron automatic missile defense control system, which calculated the interception with the issuance of data to the launch complexes of the Tal Shakar missile base. From where the two-stage solid-fuel anti-missile Hetz-3 (Strela-3) took off and brought a kinetic interceptor equipped with an infrared telescope into the interception area. The encounter with the target took place on a collision course with a convergence rate of about six kilometers per second and at an altitude slightly below 100 kilometers.
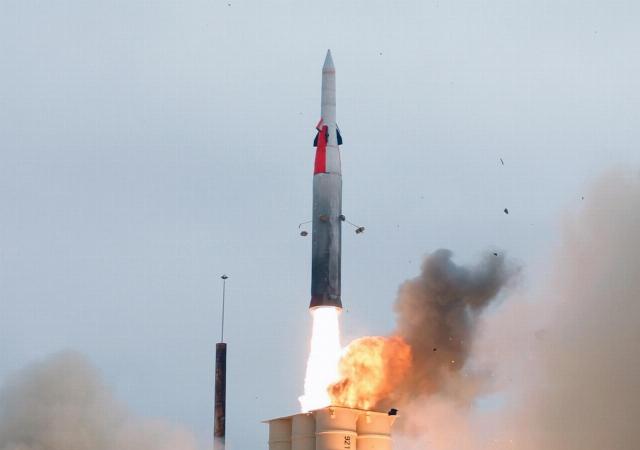
The Hetz-3 rocket. Photo: wikipedia
Many immediately called it the first combat interception in space, but the altitude data varies, estimates and 80 kilometers sound — then formally it is not a space interception.
From the point of view of the physics of the environment and the dynamics of flight for rendezvous and guidance, the altitude difference of 80 and 100 kilometers is small and does not affect interception. If this is not the first combat in space, it is still the highest altitude combat interception.
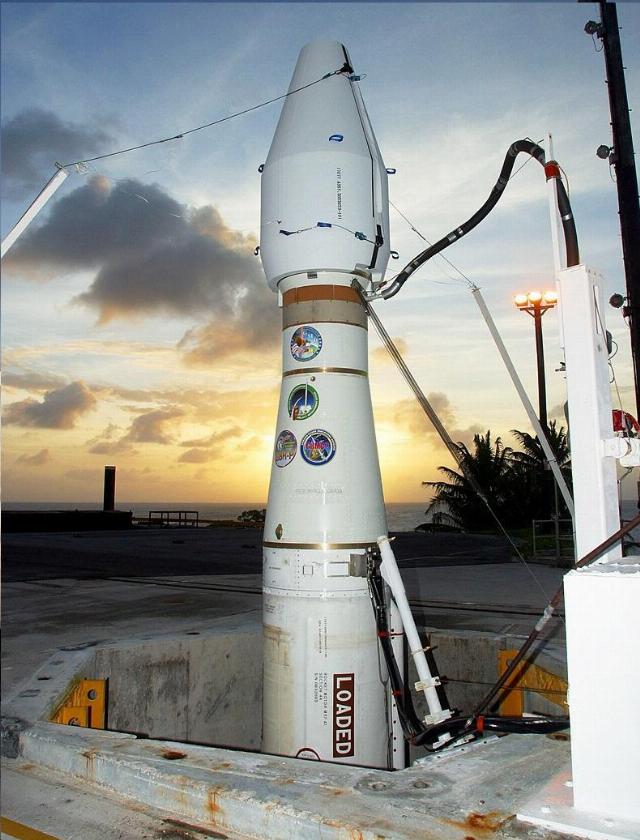
The GBI anti-missile developed by Boeing. Photo: wikipedia
And on December 12, 2023, the United States conducted a successful test interception of the atmosphere. A target missile with the parameters of the movement of medium-range missiles was launched from the air from the Hawaii area. A GBI anti-missile developed by Boeing took off from the Vandenberg base on the coast of California, part of the Western Missile Range. It is part of the ground-based missile defense system in the middle section of the GMD target flight and is on alert (and this is the fifth example of trans-atmospheric interception systems).
A three-stage solid-fuel rocket with a length of 17 meters and a mass of 13 tons has a strike height of up to 2,000 kilometers with a target distance of up to 5,500 kilometers. Its transatmospheric kinetic interceptor destroyed a target in space at a distance of 1,000 kilometers from the coast of California.
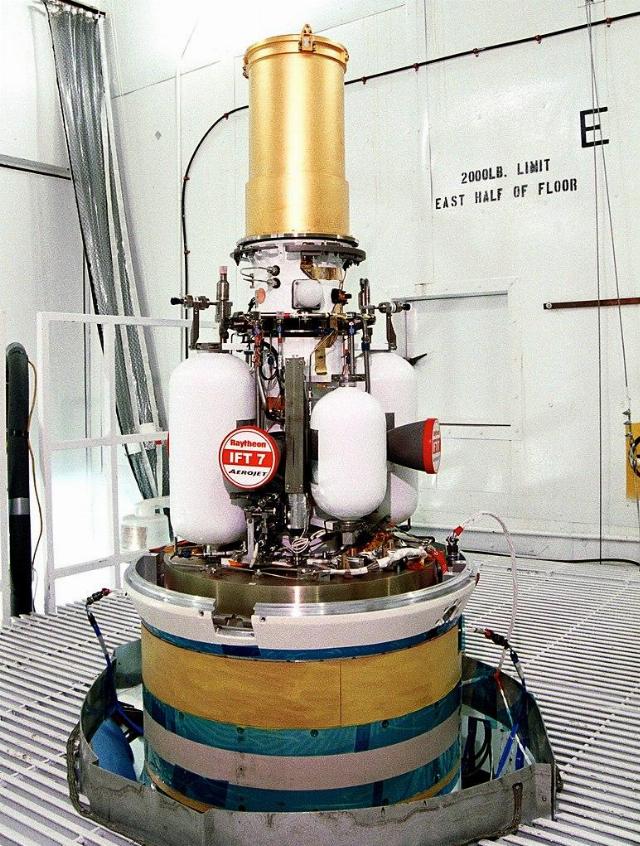
The prototype of the kinetic interceptor EKV of the GBI missile. Photo: wikipedia
This interceptor is worth a more detailed look. The EKV product from Raytheon has a diameter of 0.6 meters, a length of 1.4 meters and a weight of 64 kilograms. It maneuvers using a system of nozzles powered by compressed nitrogen, using an electron-optical guidance system with integrated backlight protection. Its speed is exceptionally high — 10 kilometers per second. In case of a counter strike on a target with its own speed of six to seven kilometers per second, the final collision speed will reach 16-17 kilometers per second.
The energy released will correspond to the explosion of a ton of TNT. It is not only sufficient to hit a target, but also vaporizes its fragments and fragments, leaving virtually no pieces dangerous to satellites. And this is another interesting approach to interception in space.
"One more last tale, and my chronicle is over."
You can dive into the issues of transatmospheric interception endlessly; the further you go, the more complicated the details, but at the same time more interesting. You can shoot down with a different choice of technical solutions, sometimes these choices are the opposite.
We tried to see the differences between the possible options, and through them — the volume and breadth of the area. The gas for the maneuvering nozzles can be hot powder and cold nitrogen. The target's observation channel is both thermal, electron-optical, and radar. The length and mass of the missiles differ tenfold; the speed of the interceptors, the height and the range of destruction at times. And all this works by embodying a variety of ideas of developers in specific products.
What's next? The development of interceptions, the development of new ideas, concepts and technical solutions continues continuously and intensively. The United States may return to the Multiple Kill Vehicle program for multiple kinetic interceptors in one missile: what was previously ineffective may become effective at a new technological level. Or with the widespread appearance of group targets — the adaptation and dissemination of technologies of separable warheads already for missiles like Scud and other operational and tactical missile systems and medium-range systems. Israel, the owner of a number of missile systems, will continue to develop missile defense.
Missile weapons that go beyond the atmosphere are becoming available to an increasing number of operating countries. Therefore, the development of protection options against it will only accelerate. The future will show which new approaches and technical systems are being implemented for trans-atmospheric interception, in which planes and facets of the task.