When a country decides to test anti-satellite missiles and shoots down its old spacecraft in orbit, the world community immediately condemns it. They say that you increase the amount of space debris for nothing and create a danger for the peaceful use of near-Earth space! Such public statements once again fuel the fear of the notorious Kessler syndrome. And one of its consequences is a widespread myth about the possibility of fighting satellites by putting a bucket of nuts (sand, nails) into orbit. However, practice shows that kinetic methods of destroying space technology are far from as effective as the military would like. Collisions of satellites with all kinds of garbage occur regularly, but they do not always fail as a result. Why? Naked Science will try to figure it out.
In March 2021, the Chinese Earth Remote Sensing satellite Yunhai-1 (02) I came across a piece of space debris. Later, retrospectively analyzing the trajectory of an unnamed "projectile" (COSPAR identifier 1996-051Q), specialists of the 18th Space Defense Squadron (18 SDS) of the US Space Forces were able to establish that this was some element of the upper stage of the Zenit-2 launch vehicle. The object remained in orbit after the launch of the Russian military satellite of radio intelligence "Cosmos-2333" in 1996. For 25 years, he lost about 60 kilometers of orbit altitude and "successfully" crossed the trajectory of Yunhai-1 (02).
The result of this incident was the formation of 37 tracked debris — this is quite a lot, considering that previous known events of this kind produced a maximum of ten new objects. The only time when more fragments turned out after the spacecraft "met" with space debris was the destruction of Iridium 33 (COSPAR ID 1997-051 C) in 2009. However, the failed Russian military communications satellite Kosmos-2251 (1993-036A) acted as garbage, so the case was rather exceptional. Both bodies were comparable in size and mass, so the result was appropriate — 2,370 fragments tracked by ground means.
But the case of Yunhai-1 (02) is not interesting at all because almost four dozen pieces of more than a centimeter in size broke off from the Chinese device. Another thing is curious — the satellite has remained operational. As far as can be judged from open sources, this fact has not been officially confirmed by the operator of the device, but there are a number of independent evidence. Judging by the historical data of the portal In-The-Sky.org, Yunhai-1 (02) has adjusted its orbit at least twice in the last year. For comparison, you can look at the orbital parameters of the part of the second stage (or payload adapter) of the rocket that launched this device into space in 2019. Being completely out of control, this object gradually loses height.
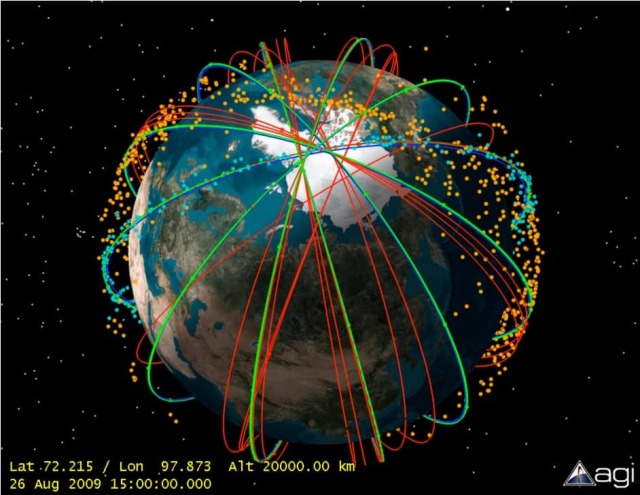
The orbits of the Iridium group satellites and the largest debris formed as a result of the collision of Iridium 33 with Kosmos-2251, as of six months after the incident
Image source: T. Kelso
Services like In-The-Sky.org they visualize in a convenient form the publicly accessible (and not very) bases of European and American near-Earth space control services. You can check the readings of such sites yourself through the appropriate resources (you will need registration) of the European Space Agency ( ESA ) and the Space Component Command of the United States, Great Britain, Canada and Australia (Combined Force Space Component Command, CFSCC ).
The performance of Yunhai-1 (02) is also confirmed by amateur astronomers observing satellites in the optical and radio bands. The device continued to transmit an encrypted data stream in the L-band (from 1 to 2 gigahertz), which was intercepted several times for analysis. After the collision, the characteristics of the radiation reflected from the satellite also changed — the frequency of brightness fluctuations disappeared. Presumably, Yunhai-1 (02) lost the antenna of the microwave radiometer and, possibly, part of the solar panels. But in one form or another, its functionality has been preserved after a collision with an object measuring from one to ten centimeters across at a speed of at least 3.5 kilometers per second.
This story is perhaps the most vivid demonstration of the very limited prospects of kinetic anti—satellite weapons. He shows that the meeting of two bodies in near—Earth space without mutual desire for it is an unlikely phenomenon and requires a fair amount of luck. And also how "tenacious" satellites are, despite the extremely impressive effects of collisions of even the smallest objects at orbital speeds. And why most of the seemingly logical methods of defeating spacecraft in space do not make sense in practice. We will tell you how bodies move in orbit, what are the difficulties in determining their trajectories and how well spacecraft are actually protected from kinetic impact.
But first, a small remark about the difficulties of understanding the processes taking place just a few hundred kilometers above our heads.
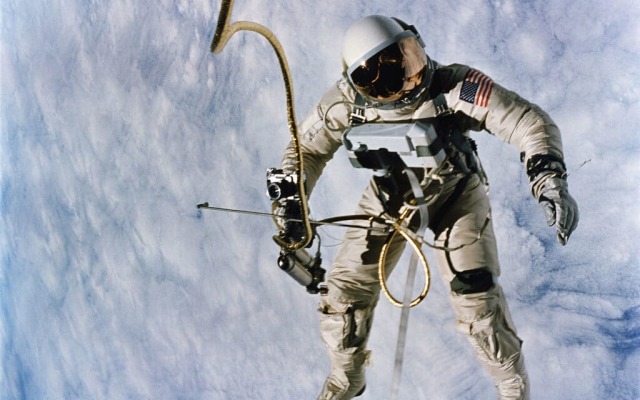
Because of the total non-obviousness of orbital mechanics, there were even embarrassments in cosmonautics. Two American pilots of the Gemini-4 mission, Edward White and James McDivitt, clearly did not complain about the lack of experience and training — the best of the best were selected for the US space program. Nevertheless, due to the fact that the task of approaching the spent stage of the Titan III rocket was set at the last moment, there was not enough time to properly prepare for it. As a result, unsuccessful rendezvous attempts cost the ship half of the fuel reserves for maneuvering engines. The pilots performed the seemingly logical actions necessary for rapprochement, but instead moved away and accelerated in completely opposite directions to the desired ones. The rendezvous then had to be canceled, but White performed the first American spacewalk perfectly
Image source: NASA, James McDivitt, US public domain
The inevitability of misunderstanding the cosmos
Everything connected with space is incredibly counterintuitive. However, as well as any areas of knowledge that go beyond the objective conditions of the human environment during its evolution. That is why it is so difficult to explain physics "on the fingers" (not necessarily even quantum). And most people's ideas about the work of their bodies have not gone far from the ancient Greeks with their four humors, the imbalance of which explains any ailments: even quite erudite people sometimes begin to talk about the "slagging" of the body. When it comes to economics or politics (and humanities), everything becomes quite difficult — the interlocutors either slide into aggressive disputes, or limit themselves to agreeing with the position "this is some kind of frivolous magic." At the same time, economics and politics are still closely intertwined with the life of a modern person.
But the cosmos is literally cut off from people — and we are not at all prepared by nature to think about it. The thinking of homo sapiens is actually "two-dimensional": you can only go forward-backward or right-left. We look up and down, but we are not able to move like that. Even when a person rises or falls, he usually moves on an inclined, but flat surface. In other words, it is incredibly difficult for us to realize the "third dimension" as a direction of free unlimited movement. Without support under your feet (in extreme cases — with your hands), or rather, a clear vector of acceleration of free fall, the human brain refuses to navigate in space.
In addition, only a hundred or a half years ago, a significant part of all people were able to move at least in a "two-dimensional" space faster than any animal. And it turned out to be incredibly difficult psychologically — the appearance of the first trains, cars and airplanes was met with creepy myths and "horror stories", like the death of passengers from the rupture of internal organs due to high speed. And it was only about speeds of tens of kilometers per hour - and in relation to devices in space, you have to realize what kilometers per second are.
Therefore, let readers familiar with orbital mechanics and physics forgive us: all further explanations and examples will be given in the most simplified version. The purpose of this article is to show the widest possible audience how huge and complex space is, even in the immediate vicinity of our planet.
"Shamanism" of orbital mechanics
Orbital motion is radically different from that on the Earth's surface or in its atmosphere. To understand it at a basic level, one must always keep in mind that any objects in orbit are constantly moving at enormous (by human standards) speeds. They are located in a space-time curved by gravity and the straight trajectories turn out to be geodesic (curves when viewed from the side). To understand the problems of anti-satellite weapons and collisions with space debris, we will try, without departing from the "two-dimensional" logic, to outline "on the fingers" the key aspects of orbital mechanics.
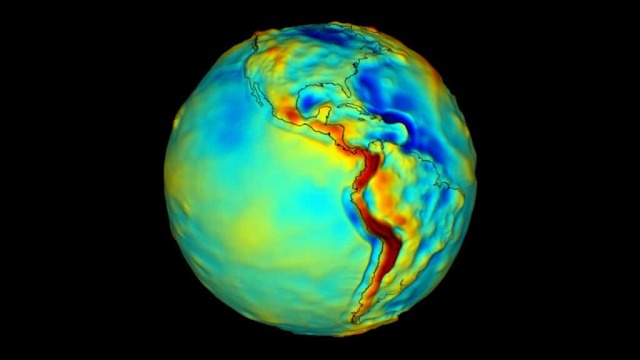
Visualization of the gravitational potential of the Earth using satellite data. Red shows the more massive areas, blue — the least. These irregularities directly affect the movement of bodies in near space
Image Source: NASA
The spacecraft moving in orbit around the planet is easiest to imagine as a freight train. He rides the rails forward and can't just turn right or left. The colossal mass interferes — a measure of inertia. Many satellites do not have as much mass, of course, as the ISS, for example, but the speed is much higher, so the inertia is still great and the energy required to change the trajectory is huge. As a result, any maneuvers are labor-intensive and time-consuming, and their effects are stretched over time. The problem of orbital motion is that the rails on which the "train" moves become an ascent when accelerating, and a descent when braking. With all the ensuing consequences: in the first case, the speed drops, and in the second case it increases, that is, everything is the opposite (acceleration slows down). A paradox.
The second important nuance, which most people forget about after school: speed and all forces are vector quantities. That is, they have not only a numerical expression, but also a direction. This means that simple arithmetic operations can be performed with them only if they are co-directed and the point of application of forces coincides. But this happens extremely rarely, so, for example, the resulting speed of movement after turning on the engines at an angle to the trajectory will have to be considered the addition of vectors (hello to the eighth grade program). Simply put, the force of inertia continues to drag the satellite straight and in order to shift to the side for some distance, its engines will need to do the work longer.
The orbit has a set of characteristics that fully describe it. They are called its Keplerian elements (because Johannes Kepler was the first to formulate them all):
- Inclination (i) is the angle of the orbital plane to a certain reference (reference plane, equatorial for planets);
- The longitude of the ascending node (Ω) is a point on the reference plane (equator) through which a body moving in orbit passes in a northerly direction (determined relative to the direction to the point of the vernal equinox);
- Eccentricity (e) — determines the shape of the trajectory (circle, ellipse or not closed);
- The semimajor axis (a) is the sum of the distances from the center of gravity to the highest and lowest points of the orbit divided in two (determines the average height of the orbit, that is, its size);
- The argument of the pericenter (ω) is the angle between the ascending node and the pericenter (determines the orientation of the orbit in its plane);
- Average anomaly (v, θ, or f for a certain epoch t0) — indicate the current position of an object moving in orbit (for example, a satellite).
Visual demonstration of the inclination of the orbit. At i = 90°, the orbit is called polar, since it passes over all latitudes at once. An inclination of more than 180 ° does not make sense — such orbits are trajectories with an inclination of 180° less, simply having the longitude of the ascending node 180 ° more (nodes change roles and places, ascending becomes descending, and vice versa). Finally, orbits with an inclination from 90° to 180° are called retrograde, since objects moving along such trajectories move against the rotation of the Earth / ©USAFA Astronautics & Space Ops, YouTube
And now the most difficult thing is to move along such rails. Suppose we don't care how the object acquired its orbital velocity (depends on altitude, for 400 kilometers — 7.672 kilometers per second), consider the ideal case. To maintain it, no action is required if there are no extraneous disturbances.
Accelerations in the forward and reverse (retrograde) directions lead to a change in the height of the orbit, as well as its shape and size (large semi-axis and eccentricity). Moreover, the point of the orbit opposite to the one in which the engines are operating rises or falls. In other words, accelerating over Africa, the satellite takes off over the Pacific Ocean (and its speed will be lower here). And then it will descend back (the speed will increase) and pass over the homeland of all mankind at the same height from which it began the maneuver (and at the speed that was before the acceleration began). The orbit turned out to be elliptical, and to return it to the shape of a circle, it will take another session of the engines at apogee. If you turn on the engines at an angle to the plane of the orbit, the inclination or longitude of the ascending node (or both) will change. Such maneuvers are the most energy—consuming of the changes in the parameters of the orbit.
The universal "currency" of any orbital maneuvers is the speed increment or characteristic speed (denoted by Δ v or ΔV, read "delta-ve"). In fact, it does not depend on the mass of the spacecraft in numerical terms, but is determined by the proportion of the working fluid for the engines of the total mass. In other words, both a thousand- and a hundred-kilogram satellite will have the same maximum possible "delta" if a quarter of their mass is occupied by fuel with the same characteristics (excluding engine efficiency).
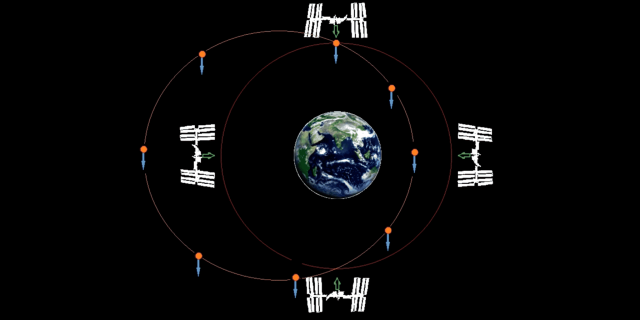
A curious effect will be obtained if you simply throw something out of the satellite "down" (or turn on its engines in this direction), that is, to the surface of the Earth. This is "something" (or the device itself) at first, indeed, it seems to approach the planet, but after a quarter of the orbit it will begin to "rise" back. By half of the orbit, the ejected object will be slightly ahead of the satellite at the same height, three—quarters of the orbit higher. And when the revolution around the Earth is completed, the trajectories will intersect at the point from which it all began. In more detail, this effect is clearly shown in the Scott Manley simulator ( video in English)
Image source: Scott Manley, YouTube
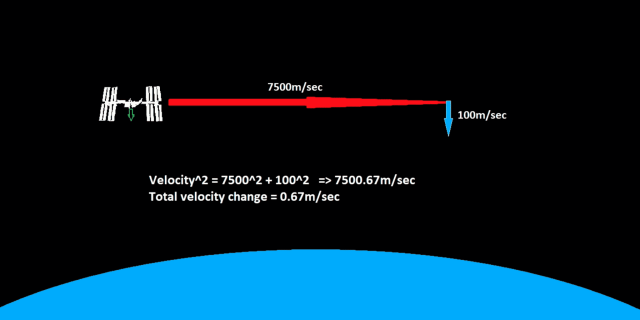
The resulting speed of such a throw will be very little different from the initial one. Although 100 meters per second of the "delta" is enough to raise the altitude of the orbit by almost 200 kilometers when accelerating in a forward direction
Image source: Scott Manley, YouTube
Hyperspeed collisions
This is another type of physical process that proceeds quite differently than intuition suggests. When it comes to collisions at speeds of tens of kilometers per hour (for example, cars) we can imagine the deformation of metal and flying debris. When a bullet meets an obstacle at a speed of several hundred meters per second, it crumples and either bounces off or passes on. But when the collision velocity is reached, which exceeds the velocity of passage of elastic waves in materials meeting each other (the speed of sound), the picture changes radically. Yes, the word "speed" is very often used in this paragraph, but it is the key to everything.
Such orders of magnitude are already called " hyperspeed " and 3 kilometers per second are considered the threshold. In such cases, at least one of the encountered bodies ceases to behave like a solid and its behavior begins to be described by the physics of liquids. Usually, a significant part of the material at the collision site evaporates, and almost all the rest of the mass passes into the liquid phase. All the subtleties of such events are still not fully understood, although active research in this area has been going on for more than half a century. Just to explain the basic nuances, a book will be needed (for example, this one ), but for our purposes, one interesting concept is enough for now — the Whipple shield .
This remarkable invention has successfully protected spacecraft in theory since the 1940s, in practice - as soon as they began to fly. The principle of operation is very simple: on the outside of the spacecraft, at some distance from the main skin, there is another thinner one. It somewhat resembles the anti-cumulative screens on armored vehicles, and the task is similar. An object flying at hyperspeed meets with a thin skin, partially evaporates and collapses, and a cloud of plasma, droplets and grains of sand already collides with the main body of the device. The energy is dispersed over a larger area. There are an incredible number of configurations of such a shield (about a hundred varieties are used on the ISS alone) for various tasks. The most important nodes of the station, for example, are reliably protected from objects up to a centimeter across.
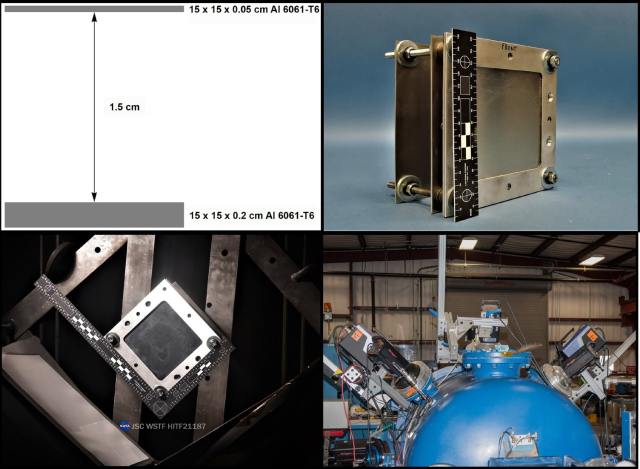
In a recent round of tests conducted by the DebriSat laboratory, the simplest configuration of the Whipple shield was used — an external aluminum plate with a thickness of 0.5 millimeters and a "main skin" with a thickness of 2 millimeters. Such a target in a vacuum chamber was fired with fragments simulating various types of space debris
Image source: NASA, DebriSat, J. Miller
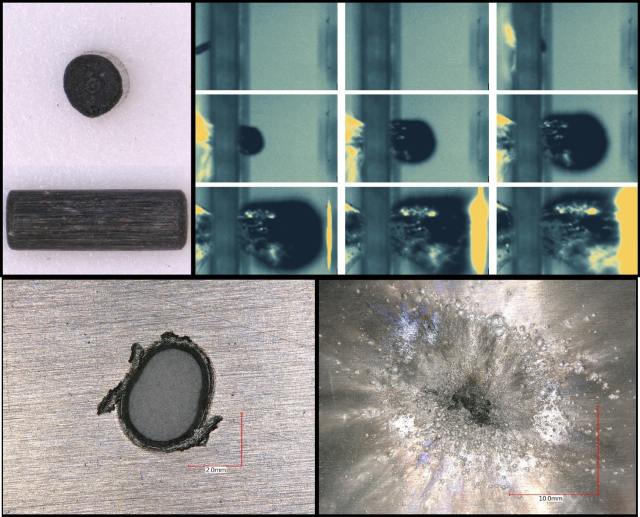
A carbon fiber cylinder (CFRP) with a length of 2.941 millimeters and a diameter of 1.029 millimeters, as expected, broke through the screen, but could not destroy the "skin"
Image source: NASA, DebriSat, J. Miller
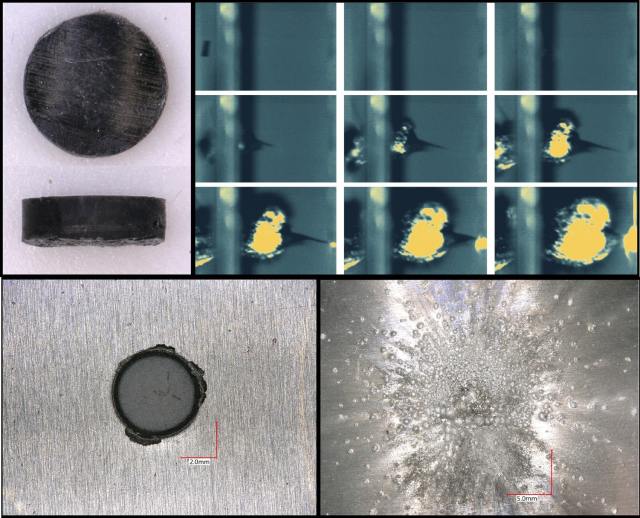
But a "tablet" made of the same material with a thickness of 0.671 millimeters and a diameter of 2.402 millimeters made a submillimeter hole in the rear wall of the target. The density of the fragments is not specified, but in the case of the "tablet" the mass was about 20% more due to the difference in volume
Image source: NASA, DebriSat, J. Miller
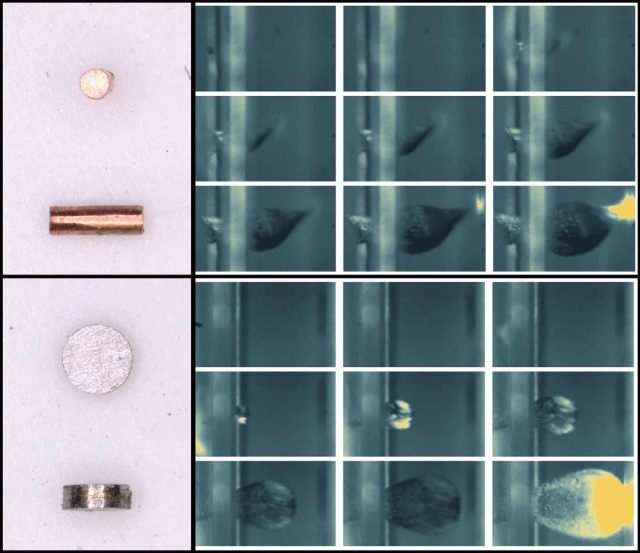
The previous two tests were performed to collect empirical data on the most common type of space debris in recent times — fragments of carbon composites. Previously, sufficient attention was not paid to them and it was necessary to check the models. For comparison, two metal fragments were additionally fired: a copper cylinder and a steel "tablet" (the masses are comparable to those for CFRP samples). In both cases, the rear wall of the target withstood the plasma cloud
Image source: NASA, DebriSat, J. Miller
And in the simplest case, the role of such a shield is performed by a heat-protective foil, which is almost always wrapped in satellites. And the most interesting property of such protection is that its effectiveness increases as the collision speed increases. Naturally, not everything is so simple and the result of a collision depends on many other parameters, of which the most significant are: the density of materials and the contact area. But speed is the main thing. Experiments to study the effectiveness of the Whipple shield are constantly being conducted and, for example, NASA regularly publishes their results ( bulletin Orbital Debris Quarterly News for the fourth quarter of 2021).
Distances
And the last thing that is most often forgotten when talking about near—Earth space is the scale. Space distances are enormous even in the immediate vicinity of our planet. Imagine that we wanted to put one spacecraft into circular orbits with a height of 300 kilometers and an inclination of 45 degrees in increments of 10 kilometers along the longitude of the ascending node. It turns out 4189 satellites — more than there are devices at all altitudes between two hundred and two thousand kilometers now. Yes, their trajectories will intersect at high latitudes, but for each one it is possible to calculate the orbital parameters so that they "diverge" from each other at a safe distance. With every ten kilometers of altitude, this number (if other conditions are maintained) will grow by six pieces.
But the following illustration speaks most clearly about the distances in near-Earth space. On it, on a scale relative to the size of our planet, the main classes of orbits by altitude are shown:
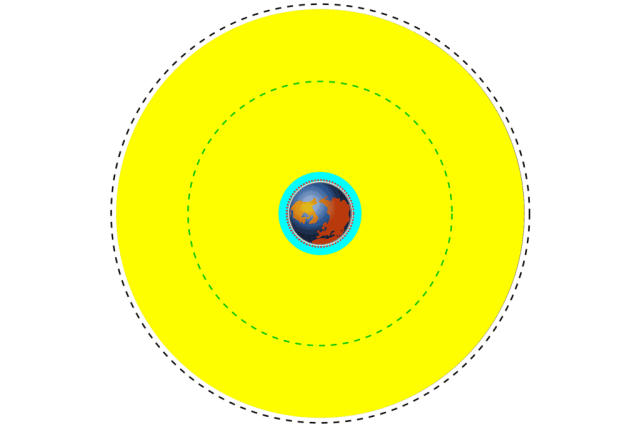
Classification of near-Earth orbits by height, scale diagram. Blue area — low (LEO), from 200 to 2000 kilometers; yellow — medium, from 2000 to 34,780 kilometers; red dotted line — the ISS orbit (about 400 kilometers); green dotted line — GPS satellites (20,230 kilometers); black dotted line — geostationary (35,786 kilometers)
Image source: Mike1024, Wikimedia
The volume of space in low Earth orbits alone (200-2000 kilometers) exceeds a trillion and a quarter cubic kilometers. And the size of satellites in this area rarely reaches a dozen meters across. Large spacecraft, with the exception of the ISS and space telescopes in LEO (low Earth orbits), are located higher. But their dimensions are still negligibly small compared to the scale of the surrounding space.
In an imperfect world
All of the above refers to certain "spherical and vacuum" conditions, the reality is even more complicated. The Earth's atmosphere, albeit very sparse, extends for more than ten thousand kilometers, and the gravitational field of the planet is heterogeneous. Depending on solar activity, the density of particles (mainly oxygen atoms, but the proportion of hydrogen and other gases is also large) in near—Earth space constantly fluctuates. This leads to uneven braking of satellites, which also depends on the cross-section of the device. A higher gravitational potential in the equatorial plane (the planet is "thicker" at the equator) results in such phenomena as the precession of the orbit and the oscillation of its Kepler elements. The full list of disturbances to which an object is subjected in near—Earth space is even greater - even light pressure and magnetic field braking are among them.
Braking during orbital motion means a loss of altitude and an increase in speed, as well as a change in the eccentricity of the orbit. Precession leads to the migration of the longitude of the ascending node in a westerly direction and the speed of this process depends on the altitude, inclination and eccentricity of the orbit. In other words, the orbital parameters are valid for a specific moment in time and change with each revolution. Moreover, without continuous observations of a particular satellite or piece of space debris, its position becomes a set of probabilities.
That is why a safety zone is designated around each man-made object in near-Earth space. For satellites on LEO — usually about a kilometer in all directions, for geostationary devices — 50 kilometers. If the trajectory of debris or another spacecraft crosses such a "sphere of uncertainty", then the approach is considered dangerous. And the satellite operator, in theory, is obliged to perform an evasion maneuver. As a rule, the altitude of the orbit simply rises by several hundred meters.
In 2018, experts in the field of near-Earth object observation named the following approximate tracking accuracy indicators: 1.5-3 kilometers for LEO, 5-10 kilometers for geosynchronous orbits. And these are American services and companies that use data from radars and telemetry stations around the world for their analytics. Ten years before, the accuracy was much lower, so the operators of the Iridium satellite constellation ignored all the warnings, considering them an unnecessary reinsurance. There are now 2370 reminders about this error (see above).
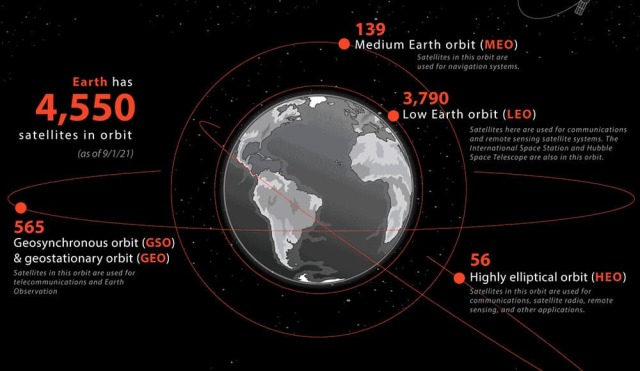
A fragment of the infographic "Every satellite in Earth orbit and who owns them" from the DEWESoft portal as of January 18, 2022
Image Source: DEWESoft
A lot has changed since then and new surveillance centers are being built every year. Moreover, not all of them are state—owned - the growing trend towards the commercialization of space makes such services extremely in demand. So separate companies create their own radars and multispectral trackers both for their own needs and for the purpose of selling data.
And how to shoot them down?
Let us summarize all of the above in relation to the defeat of a simulated enemy spacecraft. This task is divided into two subtasks, each of which is a real engineering and scientific adventure:
- Identification of the target and refinement of its orbital parameters;
- Performing an interception.
Some orbital parameters are already there, it remains to clarify them. In fact, this happens simultaneously with identification. Thus, by measuring the Doppler effect for the signal emitted by the device, its speed is determined. But the most difficult thing is to reduce the measurement error of the orbital parameters of the target to at least hundreds of meters. The problem is that even the most sensitive radar on the Earth's surface will receive few photons reflected from the satellite. And according to this weak signal, in a few minutes (the time of passage of the orbital arc over the observation site), the tracking station should issue a target designation.
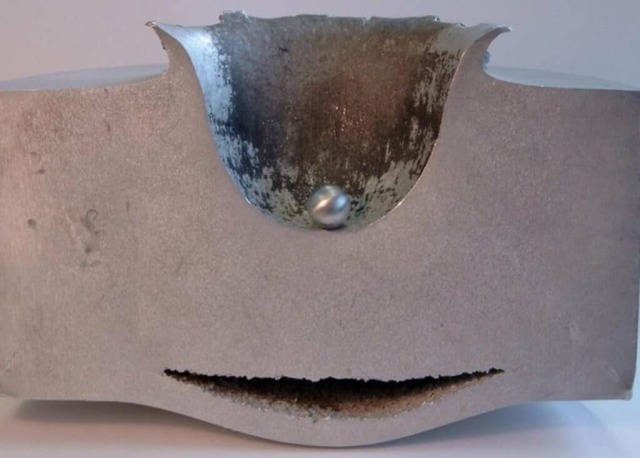
The dangers of space debris and collisions at hyperspeed are often illustrated with similar photos. On which a small projectile does truly amazing things with all-metal targets. Nevertheless, if there were another screen of almost any thickness in front of this block of aluminum, the effect would be much less impressive
Image Source: ESA
Let's assume that open catalogs of near-Earth objects are available and you and I have the opportunity to find the satellite of interest in them (or we have our own fairly complete database). Let's leave aside the question of choosing a goal — these are military problems, we are only interested in the technical side. But we still have to make sure that a particular object in the sky is exactly what we need. To do this, it will be necessary to use means of electronic intelligence (interception of transmitted data, comparison of radiation signatures), at least, and even better, optical methods (that is, a telescope).
What determines the resolution of radars is a topic for a separate book. But in our case, the main thing is that the longer it takes to observe the goal, the better. Ideally, at least a couple of turns are needed, but only a few satellites on LEO pass over the same place once a day. And here we smoothly approach the need to have several observation stations in different hemispheres. There is no way to do without special ships of the measuring complex, but as of 2022, only China and the United States have enough of them. Russia after the collapse of the USSR all such vessels html" target="_blank" rel="nofollow">sent to a museum or for scrap, except for "Marshal Krylov". And only the United States, together with partners (EU, Canada, Australia), has a developed network of ground-based radar complexes and telescopes for such purposes.
Let's say the target was identified, identified and found. What to shoot down? If we discard lasers, then the choice is small. The means of destruction can be ground-based or space-based. The trouble with the latter is the need to put the interceptor into orbit in advance with characteristics close to the characteristics of the target orbit. That is, it will not be possible to do it unnoticed, and the maneuvering space for such a device is small. Why this is so is described above in the section about orbital mechanics. If the interceptor can still "play" with the height and shape of the orbit within a fairly large range, then it will take a lot of fuel to change the inclination by more than a couple of degrees. But there is also the longitude of the ascending node.
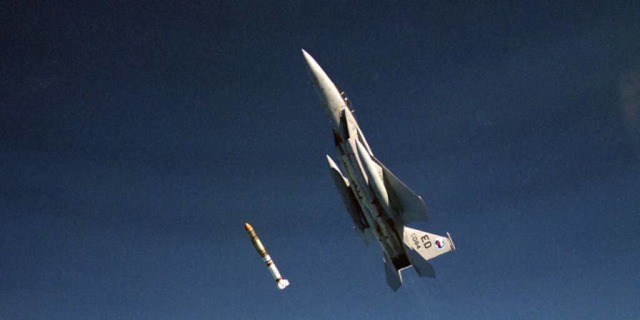
Test launch of the ASM-135 ASAT anti-satellite missile from an F-15A fighter on September 13, 1985. A self-guided warhead (MHV) with a total mass of 14 kilograms successfully hit the Solwind P78-1 satellite. The collision occurred at an altitude of 555 kilometers, the speed reached 6.7 kilometers per second
Image source: Paul E. Reynolds, US public domain
Nevertheless, projects of such devices existed, and some of them were successfully implemented in metal. A striking example is the Soviet " Satellite Fighter " (IS). His exact characteristics have never been published in open sources, it is only known that he was able to change not only the height and eccentricity, but also the inclination. However, it is not specified within what limits. The Turaev Machine-building Design Bureau "Soyuz" indicates the delta reserve for the IC at 1200 meters per second. This is enough to change the inclination by 8 degrees for a circular orbit with a height of 400 kilometers (or raise its height to more than 3000 kilometers), but the reliability of this information is questionable.
Earth—based weapons of destruction are anti-satellite missiles. They are launched on a suborbital trajectory so as to meet the target at a minimum distance. It turns out that their launchers (or airfields, if missiles are launched from airplanes) must be in a certain proximity to the route (projection of its orbit to the Earth's surface) of the affected satellite. Otherwise, the interception will simply not take place. But there is a surprise factor — it will take a few minutes from the launch of the rocket to the hit. But such a "shot" will not be cheap: high energy is needed to throw a warhead a thousand kilometers up, and the head itself must necessarily be maneuverable. There is still no way to get rid of the error of "aiming" in kilometers. Moreover, in order to maximize the chance of defeat, the homing head must be multispectral (for example, an infrared sensor and radar).
Weapons of destruction and space debris
Both of these issues are closely related. The choice of the method of inflicting damage to an enemy spacecraft depends to the greatest extent on the ability of the entire anti-satellite complex to determine the orbital parameters of the target. In the most advanced case, a thermonuclear charge will be needed, striking with infrared, X-ray and neutron radiation (there is no medium outside the atmosphere for the formation of a shock wave). However, even for a megaton of TNT equivalent, it is desirable to be at a distance of no more than a couple of kilometers from the target. If the warhead can explode tens of meters from the victim, then a fragmentation warhead will do. The possibility of forming a beam of fragments increases the permissible error, but it is necessary to ensure sufficient density of damaging elements.
We will have to dwell on this point in a little more detail. The fact is that space debris is actually the same shrapnel. And its fragments measuring more than 10 centimeters, humanity has already learned to track with sufficient accuracy to prevent collisions with them. At least, in the case when the satellite operator uses the services of European and American near-Earth space monitoring services, and also listens to their warnings about dangerous approaches. Garbage in the range from a centimeter to ten is already a problem, even smaller sizes are not traceable at all. Therefore, most long-lived spacecraft are adapted to survive a collision with such objects. Critical systems are shielded, carried in the design and duplicated, both physically and programmatically.
Guaranteed damage to the satellite can either be an object larger than a centimeter across, or smaller, but met with it at a speed of less than three kilometers per second (and then if it successfully hits something "vital"). This creates big problems for developers of anti-satellite weapons. Or you will have to hit the target with a monolithic impactor, but this will require an incredibly high accuracy of guidance. Or "shoot" fragments "in pursuit". There is an option to equip the warhead with striking elements ranging in size from 1 to 10 centimeters, but then it will have a large mass.
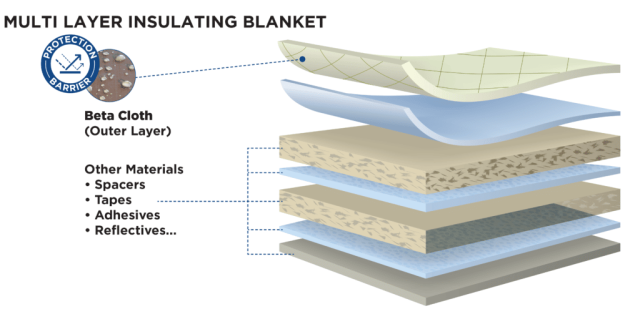
The structure of a sheet of typical multilayer thermal insulation (MLI, screen-vacuum insulation) of spacecraft. It consists of alternating sheets of reflective (mylar or kapton with a metal coating) and filling materials (mineral wool, mesh, gas weave fabric) with one or more particularly strong layers (beta fabric). In fact — a ready-made Whipple shield with filler
Image source: Saint-Gobain
It immediately becomes clear how ridiculous the idea of throwing a bucket of nuts, nails or sand into orbit is. Of course, they will damage the protruding elements of the satellite like solar panels, antennas and some sensors. But only if they meet with the device immediately at the moment of scattering from a hypothetical bucket. A few hours after removing the nuts or nails (we will not consider the sand at all because of the complete meaninglessness) will begin to diverge and randomly distributed. The density of their cloud will be insufficient even to properly "rip off" one satellite, not to mention a full-fledged Kessler syndrome at a particular altitude of the orbit.
But there is also the cost of bringing the required number of striking elements into the target orbital planes. Of course, you can always try to "play" with the material of conditional "nuts and nails". The same is true for the fragmentation warhead of an anti-satellite missile. To make them, for example, from carbon composites or tungsten — in other words, to increase the evaporation temperature of the damaging elements. Thus, it will be possible to raise their danger to space technology somewhat, but the cost will increase dramatically. In any case, the chances of satellites meeting with these nails and nuts will still increase slightly. And the hopes for a cascade effect with a multiplication of the number of fragments are somewhat overstated — the formation of many new objects during collisions at hyperspeeds is unlikely (objects must have a comparable mass or a complex shape)
The only way to guarantee a critical threat to all vehicles in low Earth orbit is to create a man—made ring of sufficiently large striking elements in the equatorial plane (it is crossed by any satellites). How many of them will be needed for a disk with a width of 1800 kilometers with a density of at least one "nut" per square meter, we suggest readers to calculate on their own, our calculator failed.
Then why is it necessary
It turns out that kinetic anti-satellite weapons are too complex and expensive to defeat satellites. It can guarantee destruction only if a number of conditions and a surprise attack are met. Nothing prevents an apparatus that risks becoming a target from chaotically changing its trajectory by a kilometer or two in wartime. Yes, his life span will be drastically reduced, but it can be justified. Satellites are now getting cheaper (and smaller), and the means of their removal are becoming more operational. However, any anti-satellite missile is not actually tested against satellites. First of all, it is an indispensable part of protection against intercontinental ballistic missiles. And in this role, kinetic interceptors have no equal yet.
Finally, about Kessler syndrome and garbage
If you have not yet heard about Kessler syndrome, then you can briefly familiarize yourself with it here . Of course, half a century ago, when the means of tracking objects in near-Earth space had extremely low resolution, such concerns made sense. But modern satellites are able to maneuver, when designing them, the scenarios of information from orbit or burial are taken into account. This, in general, radically reduces the littering of space. In addition, monitoring and timely notification allows you to avoid collisions. If, of course, the operator works in international cooperation. Apparently, Yunhai-1 (02) has become a victim of politics — it is a device, presumably, dual-use and the Chinese side was denied the use of American-European catalogs. Or in the Middle Kingdom they themselves decided not to connect to them.
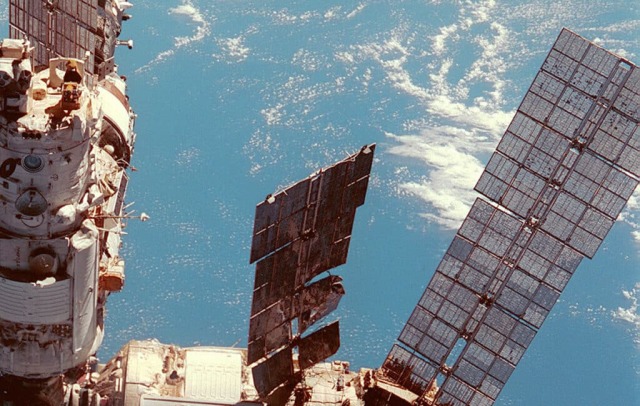
The Mir space Station has experienced a considerable number of collisions with space debris and micrometeoroids. The large solar panel of the Spectrum module on the right side of the image is speckled with their traces. The smaller panel in the center of the image was damaged during the collision with the Progress M-34 spacecraft. Photo taken in June 1998
Image source: NASA, Crew of STS-91
The private space company SpaceX alone has launched more satellites over the past few years than most countries have in previous decades. And all these devices, with some exceptions, work. And those that are not — smoothly and controllably descend into the atmosphere. With proper management of such a "constellation", the probability of serious incidents in orbit is vanishingly small. And those that happen rarely remain noticed by anyone other than the satellite operator. Because damage almost never becomes critical. Maximum — several the cells of the solar battery will knock out or leave a spectacular mark on the skin .
An attentive reader may immediately notice that the general soothing tone of our article contrasts sharply with the widespread alarmism about space debris. Take at least an information campaign The European Space Agency (ESA) or the reaction of the international community to the Russian tests of anti-satellite weapons. On the one hand, this is due to the difficulty of understanding all the subtleties related to this topic (we hope this text helped clarify them). On the other hand, completely different approaches to risk assessment are applicable in different situations. Yes, space debris is a problem, albeit a little exaggerated. But it is better to react to it in this way than to ignore it altogether.
As for the ISS and the explosion of Kosmos-1408 last fall, the alarmism of that time was just clear: the minimum distance between the calculated orbits of the fragments and the station's security sphere was only 40 kilometers in height. This is very small — tens of meters per second of "delta" (throws in baseball are more powerful ). Could anyone in the first days after the tests guarantee that no fragment received sufficient acceleration for such a decrease? No, and it is physically impossible to confirm this without long-term observations. And in the case of the ISS, any collision is considered dangerous, regardless of the size of the object.